Nonlinear Quantum Optics
Dispersion-Enabled Tools for Quantum State Engineering
Integrated devices are not merely micron-scale equivalents of their bulk-optics counterparts. Their inherently dispersive characteristics can be exploited to unlock new capabilities, allowing them to play a remarkably more versatile role in quantum circuit architectures. We have shown this by examining the implications of linear dispersion in an ordinary directional coupler. Coupling dispersion unlocks several novel capabilities for this device, including in situ control over photon spectral and polarization entanglement, tunable photon time ordering, and entanglement-sensitive two-photon coincidence generation. It also becomes possible to maintain perfect two-photon anti-coalescence while tuning the interference visibility, which has no equivalent in bulk optics. Our work adds to a suite of state engineering and characterization tools that benefit from the advantages of integration. It also paves the way for re-evaluating the possibilities offered by dispersion in other on-chip devices.
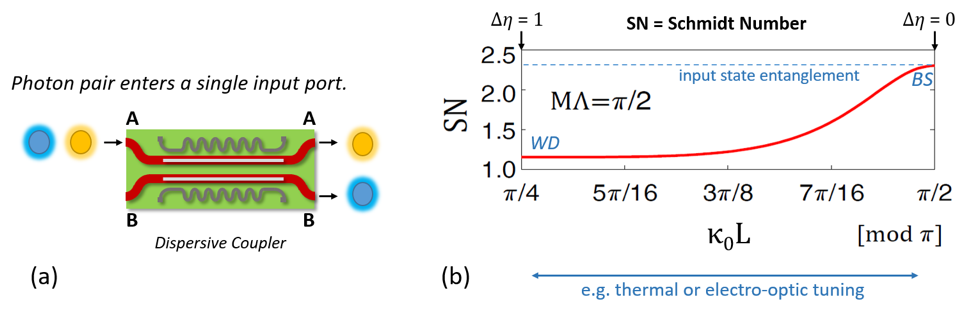
Figure 1: Figure 1: Tuning post-selected spectral entanglement via couper dispersion.
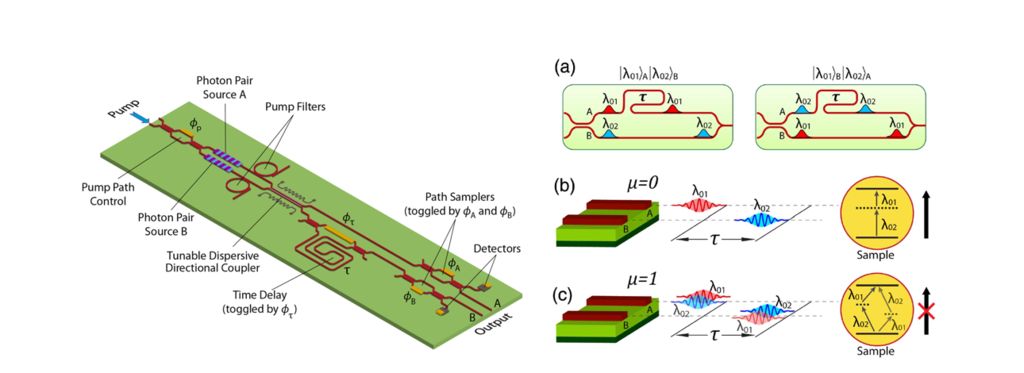
Figure 2: Tunable time-ordering for control over two-photon absorption pathways.
Interference-Facilitated Photon Pair Separation
Interference-Facilitated Photon Pair Separation
Many quantum protocols and applications require newly-generated photon pairs to be separated into different spatial paths, so that each may be manipulated independently. This can present difficulties for integrated on-chip sources (Fig. 1) due to the drawbacks of conventional separation techniques. Some techniques (e.g. wavelength de-multiplexing) only work for certain states and degrade entanglement, while others (e.g. probabilistic separation though a 50:50 beamsplitter) induce a 50% loss of photon flux. This problem is solved by Interference-Facilitated Pair Separation (IFPS), which uses the quantum interference of two indistinguishable generation pathways to deterministically separate any arbitrary bunched two-photon state without adverse impact on entanglement properties.
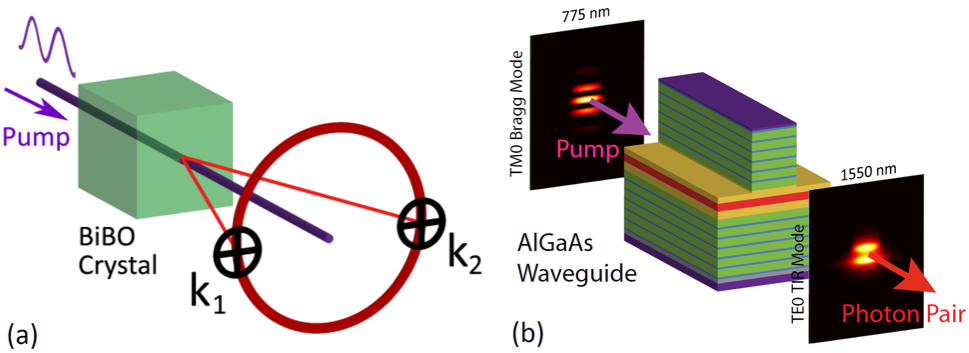
Figure 1: (a) Separation in free-space bulk-optics is straightforward because the photons are always found at opposite (antipodal) points of a cone. (b) In contrast, twin photons generated in on-chip structures often overlap and co-propagate within the same integrated waveguide.
Given the universality of IFPS and its utility for quantum light sources of high tunability, it is important to understand how integrated device dependencies, such as coupler dispersion and birefringence (i.e. dependence of the coupling strength on wavelength and polarization), impact its behaviour. We found that IFPS performance depends not only on the linearity of the coupling dispersion but also on the photon bandwidth in addition to spectral entanglement. As the photon pair non-degeneracy is tuned, any nonlinearity present in the coupling dispersion leads to a degraded separation fidelity. This, however, can be mitigated by tuning either the overall coupling strength or the phase-matched degeneracy wavelength of the photon pair source itself. On the other hand, when the coupler dispersion is perfectly linear, symmetry in the coupler de-multiplexing behaviour can compensate for the loss of quantum interference, resulting in maintaining a perfect separation fidelity. Our findings aid the design of devices for universal photon pair separation of two-photon states with tunable properties.
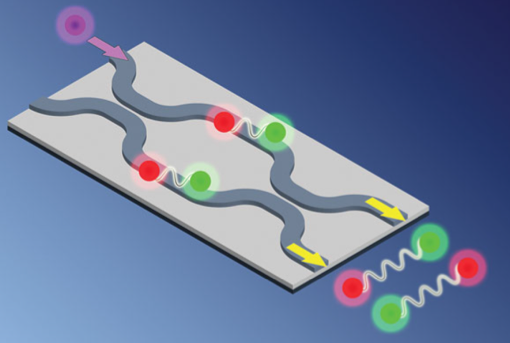
Figure 2: Depiction of interference-facilitated pair separation (IFPS). The pairs are in superposition.
Multiple Polarization Entangled Sources from the same Monolithic Semiconductor Waveguides
Multiple Polarization Entangled Sources from the same Monolithic Semiconductor Waveguides
Generating nonclassical states of photons such as polarization entangled states on a monolithic chip is a crucial step towards practical applications of optical quantum information processing such as quantum computing and quantum key distribution. Here we demonstrate two polarization entangled photon sources in a single monolithic semiconductor waveguide. We show AlGaAs based Bragg reflection waveguides (BRWs) can be used to simultaneously produce two polarization entangled photon sources using alternative approaches in a single self-contained, room-temperature semiconductor chip.
The first source of polarization entangled photon is achieved via two concurrent spontaneous parametric down-conversion (SPDC) processes. Those two processes with a shared TM polarized pump, paired photons can be either generated in TM polarizations via the type-0 process, or in TE polarizations via type-I process. The concurrent phase-matching of those two SPDC can be achieved by engineering the structure of BRWs. In the ideal case, photon pairs can be produced with the same efficiency and identical spectrum, which renders them in a maximally entangled state (|H,H⟩+e^iϕ |V,V⟩)/√2 . The same chip can also be used as a source to generate polarization entangled photons via the type-II SPDC process. The phase-matching wavelength of type-II is only a few nanometer below those of type-0 and type-I. We perform a variety of correlation tests on the photon pairs and show the output quantum state of both source has a high quality of entanglement.
We demonstrate that the device has capability to have three types of phase-matching simultaneously and allows the generation of both co-polarized and cross-polarized entangled photons pair. In both cases, polarization entanglement is generated directly on the chip without the use of any off-chip compensation, interferometry or bandpass filtering. This enables direct, chip-based generation of both Bell states (|H,H⟩+|V,V⟩)/√2 and (|H,V⟩+|V,H⟩)/√2 simultaneously utilizing the same pump source. In addition, based on compound semiconductors, this chip can be directly integrated with its own pump laser. This technique ushers an era of self-contained, integrated, electrically pumped, room-temperature polarization entangled photon sources.
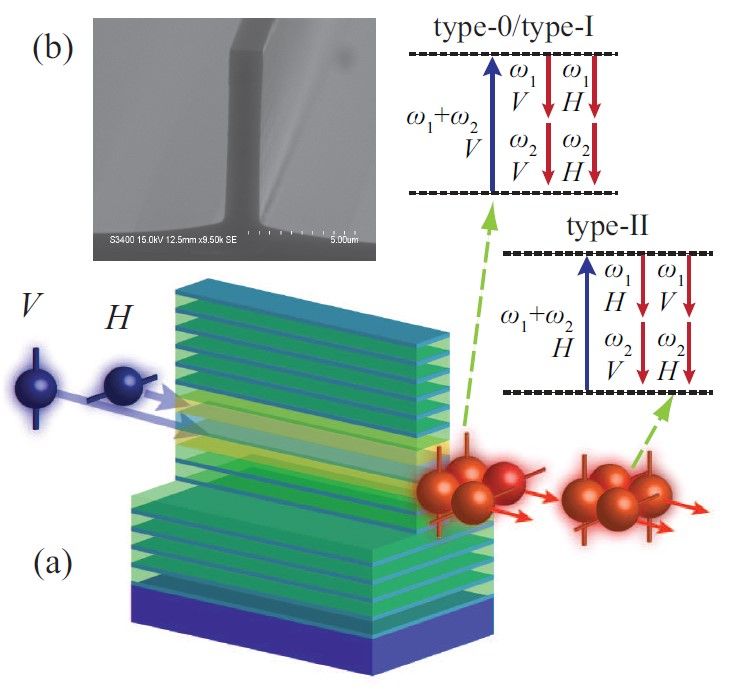
Fig.3 TM pump generate co-polarized entangled photon pair via type-0 and type-I process. TE pump generate cross-polarized photon pair via type-II process.
Hyperentangled sources in Semiconductor Waveguides
We propose and analyze the performance of a technique to generate mode and polarization hyperentangled photons in monolithic semiconductor waveguides using two concurrent type-II spontaneous parametric down conversion (SPDC) processes.
Paired photons are generated by utilizing phase matching in AlGaAs Bragg reflection waveguides (BRW). We show that two type-II SPDC processes are phase matched at the same operating wavelength by engineering the structure of the BRW. As shown in Fig.2 a), as the ridge width decrease, the effective index of the pump mode decreases much faster than those of the generated photon modes. The birefringence of BRWs can be tuned by changing the core thickness. The concurrent phase matching is achieved by tuning both ridge width and core thickness of BRWs.
Paired photons generated in each process are cross polarized and guided by different guiding mechanisms, which produces entanglement in both polarization and spatial mode. The first SPDC process generates a photon in a TE Bragg mode and one in a TM total internal reflection (TIR) mode, while the second SPDC process generates a photon in a TM Bragg mode and one in a TE TIR mode. The spatial modes with different guiding mechanisms open a way to generate hyperentanglement making use of the spatial degrees of freedom (DOF).
Theoretical analysis shows that the degree of entanglement increase as the filter bandwidth decreases. The output quantum state has a high quality of hyperentanglement by spectral filtering with a bandwidth of a few nanometers, while off-chip compensation is not needed. This technique offers a path to realize an electrically pumped hyperentangled photon source.
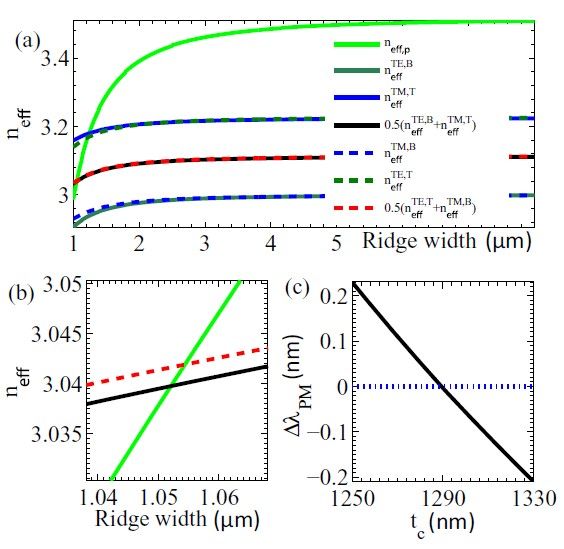
Fig.2 a)b) Dependence of effective index of each mode on ridge width. c) difference of PM wavelength of two SPDC as function of core thickness
Spectral Engineering of Photon Pairs
This work proposes and analyses a novel approach for the generation of separable (quantum uncorrelated) photon pairs based on spontaneous parametric down-conversion in Bragg reflection waveguides composed of semiconductor AlGaN layers. This platform allows the removal of any spectral correlation between paired photons that propagate in different spatial modes. The photons can be designed to show equal or different spectra by tuning the structural parameters and hence the dispersion of the waveguide.
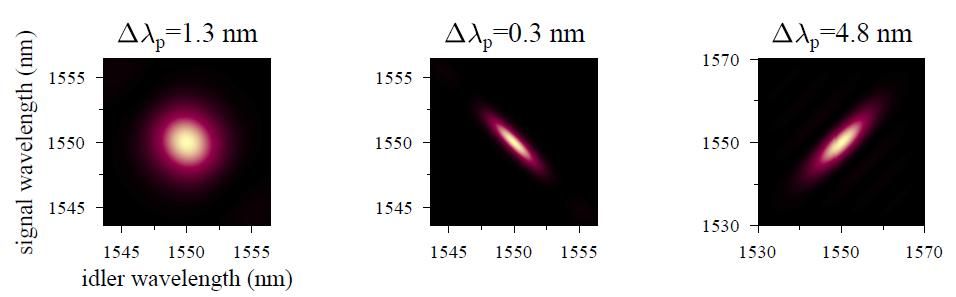
Joint spectral intensities of photon pairs generated by the proposed structure with different pump spectral bandwidths.
Bandwidth Control of Paired Photons Generated in Monolithic Bragg Reflection Waveguides (link)
We consider Bragg reflection waveguides as monolithic sources of frequency correlated photon pairs generated using spontaneous-parametric down-conversion in a AlxGa1−xAs material system. The source offers unprecedented control over the process bandwidth, enabling bandwidth tunability between 1 nm and 450 nm while using the same wafer structure. This tuning is achieved by exploiting the powerful control over the waveguide dispersion properties afforded by the phase-matching technique used. The offered technology provides a route for realizing electrically pumped, monolithic photon pair sources on a chip with versatile characteristics.
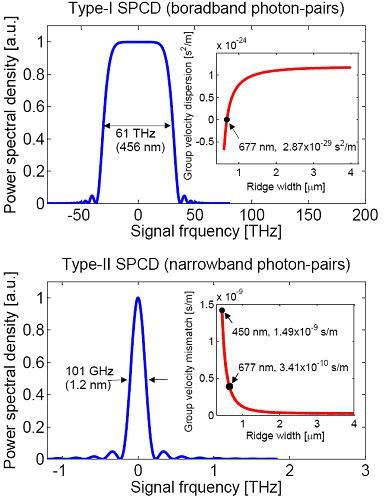
Broad and narrow SPDC spectra produced by Bragg reflection waveguides with different ridge widths.